Alain Ambrosi (Canada) is a designer and producer of intercultural projects, independent researcher, author, videographer and producer of the Remix The Commons Project.
Patterns of
COMMONING
OpenSPIM: A High-Tech Commons for Research and Education
By Jacques Paysan
Scientific and medical research critically depends on being able to observe very small structures that are invisible to the naked eye. Neuroscientists seeking to find cures for injury-induced paralysis, for example, may want to be able to observe the axons of regenerating neurons on coated nanowires. This kind of experiment often requires extremely high-powered and sophisticated microscopy. While demand for high-end, innovative microscopes is significant, there is only a handful of companies in the world that manufacture such instruments. One reason for this is certainly that the production of high-quality optical equipment in larger scales requires levels of precision and sophistication that are difficult to reproduce. It seems that the smaller the object being observed, the bigger the problems become. The effect of any aberration in the optical system is enlarged along with the desired information. A disturbance of only one thousandth of a millimeter can lead to visible abnormalities. Thus, even with detailed technical knowledge, the manufacturing of high-precision microscopes remains an enormous challenge.
It is therefore a remarkable development that this high-tech domain has recently been invaded by a project, OpenSPIM, in which researchers and engineers are cooperating on all levels under a regime of commons principles. The projects show the power and effectiveness of networked cooperation at the highest levels of scientific research and precision manufacturing.
SPIM is an acronym for “Selective Plane Illumination Microscopy,” which is also known by a more intuitive name, light-sheet microscopy. SPIM differs from other microscopic technologies in how it illuminates observed objects. In conventional instruments, the sample is usually illuminated along the optical axis,1 either through a lens underneath the object or through the microscope objective in the viewing direction. In light-sheet microscopy, however, the illumination light traverses the object like a thin sheet from one side to the other, perpendicular to the optical axis and not from below or above. This unusual configuration gave rise to the technology’s name – selective plane illumination.
This illumination technique was first developed in the beginning of the twentieth century by Richard Zsigmondy and Henry Siedentopf in their so-called Ultramicroscope. When Zsigmondy was awarded the Nobel Prize for Chemistry in 1925, Professor Söderbaum described this instrument quite nicely in his presentation speech:
The idea originated from Zsigmondy and was developed in detail by him in cooperation with Siedentopf, an able optician with the firm of Zeiss. The principle of this instrument is briefly that the intensely illuminated object, the solution to be examined, is observed by means of a microscope from the side, i.e., vertically to the axis of the incident light beam. In this way it is possible to differentiate between particles of such small size that they could not be observed under an ordinary microscope, just as the dust particles suspended in the air in our rooms, which are invisible under ordinary conditions, sometimes become visible when the sun’s rays shine through the window in a definite direction in relation to the observer.2
For understanding the significance of the SPIM principle, it might be helpful to recall some basics of light microscopy. In transmitted light microscopy, nontransparent structures become visible because they absorb light and therefore appear darker. In contrast, reflected light microscopy makes objects visible through a reflection or scattering of light, which makes them brighter. Fluorescence microscopy can be regarded as a special case of reflection microscopy, where the illumination light is used to cause observable fluorescence of specific structures in the specimen under investigation. Fluorescence microscopy has grown dramatically in recent decades, especially with the invention of a variety of new biogenic dyes (e.g., Green Fluorescent Protein (GFP) and its spectral variants), letting researchers now observe processes in living cells, in whole organs, or even in intact living organisms.
The problem with this very powerful approach is the high photon density that is required to cause fluorescence in the sample. During an observation, the sample is exposed to such intense excitation light that dye molecules soon bleach away and the observed samples are damaged or even die. This damage gets particularly severe because the excitation light beam has to travel along the optical axis through the entire specimen; in three-dimensional objects, this destructive flood of photons needs to be repeated for each level of observation.
By using an elegant trick, however, SPIM revolutionizes fluorescence microscopy exactly at this point: The microscope is able to illuminate precisely and exclusively the plane of the object that one wishes to observe. Other parts of the object remain untouched by the intense ray of excitation light, and the points above or below the plane of focus are not even elevated to a glow.
Thus SPIM can actually produce an “optical sectioning” enlargement of thick three-dimensional samples “on the fly.” This is something that traditional “confocal microscopy” can accomplish only through an elaborate point scanning procedure. SPIM avoids this process by illuminating the entire plane of focus, which can now be imaged in milliseconds using high-speed digital cameras. This process also makes it possible to gently observe intact living samples for hours or even days and from multiple perspectives, without destroying the sample. Consequently, SPIM is typically used to observe insect larvae, zebrafish embryos, the growth of tumors, organoids, and regenerating nerve fibers. Because of its versatility, SPIM has quickly attracted a growing community of enthusiastic followers among researchers interested in noninvasive microscopy on living organisms.
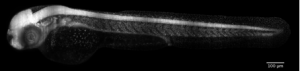
Figure 1: An OpenSPIM image of a 48-hour-old, living zebrafish embryo, in which certain structures like the nervous system and cell nuclei are labeled with the Green Fluorescent Protein (GFP). Photo by openSPIM, under a Creative Commons BY-SA 3.0 license, via https://openspim.org/Welcome_to_the_openSPIM_Wiki.
Globally, an estimated 100 SPIM systems had been “homebuilt” by this community before ZEISS introduced the first commercial light-sheet microscope in October 2012. Two years later, members of the SPIM community and curious researchers from all over the world converged on Barcelona to attend the First International Lightsheet Fluorescence Microscopy conference, where they eagerly exchanged experiences and ideas for applying and improving SPIM.
Among the speakers at this conference were Ernst Stelzer and Jan Huisken, who are regarded as key inventors of modern SPIM. The reanimation of the old oblique illumination principle known as SPIM in modern research drew upon academic research in which Huisken was significantly involved as a graduate student in Ernst Stelzer’s lab, at the renowned European Molecular Biology Laboratory (EMBL) in Heidelberg, Germany. As a postdoctoral researcher at the University of California, San Francisco, Huisken later continued to adapt SPIM to biological applications before returning to Germany to start his own research group at the Max Planck Institute for Molecular Cell Biology and Genetics in Dresden (Germany), where he obviously inspired a creative nucleus for further SPIM-related ideas.
It was there where the next breakthrough occurred: “OpenSPIM started, once upon time, at the institute’s canteen where they dreamed up SPIM in a suitcase,” as his colleague Pavel Tomancak tweeted. “It actually exists!” Supported by the international Human Frontiers Scientific Program, the idea was first developed through a wiki,3 which, consistent with the basic ideas of the commons, was published under a Copyleft (CC BY-SA 3.0) license.4 In this wiki, the interested researcher can find a precise list of necessary parts, assembly instructions and video tutorials describing how to build a ready-to-use OpenSPIM instrument in less than one hour, in fourteen discrete steps. From the beginning, the OpenSPIM project committed itself to the principles of open hardware and open software (Pitrone et al. 2013).
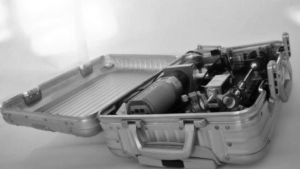
Figure 2. OpenSPIM in a suitcase. The camera is seen in the bottom left, the sample chamber in the upper left corner. The box in the center is the laser light-source from which the blue laser is reflected by several mirrors into the sample chamber. Photo by OpenSPIM, under a Creative Commons BY-SA license, 3.0, via https://openspim.org/Gallery.
To fully appreciate the achievements of this project, one needs to understand the complex and challenging data-analysis requirements of SPIM microscopy. Depending on its configuration, a SPIM microscope can generate more than 100 megabytes of data per second, potentially during the course of an entire week, all of which must be handled, stored, analyzed and rendered. Simply storing such vast quantities of data, where individual datasets can comprise up to several terabytes, is prohibitively costly for normal computing systems. The system often requires specific software that can work on cloud computing platforms, i.e., high-performance computing on distributed computer networks. Performing such computation at acceptable speeds on individual machines is highly problematic.
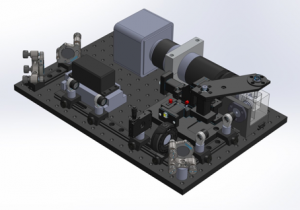
Figure 3: The completed OpenSPIM as shown in the open assembly instruction. Photo by OpenSPIM, under a Creative Commons BY-SA license 3.0, via https://openspim.org/Step_by_step_assembly.
Fortunately, the open structure of OpenSPIM provides a solid groundwork for mastering these challenges. For example, OpenSPIM uses only open source Arduino electronic components, and the operation of the system is controlled by the free software µManager. Data analysis and rendering are managed by specialized plugins to the software package Fiji/ImageJ, which is an extensive open source software project for the analysis and processing of scientific image data. Much of the work for those plugins has come from a team led by Pavel Tomancak, a congenial Czech who works at the Max Planck Institute in Dresden. This group is not only renowned as a driving force of the OpenSPIM project, but is highly respected for its missionary zeal in hosting workshops, conferences and academic collaborations to share its knowledge within the growing OpenSPIM community.
Since OpenSPIM started two years ago, the design plans for seven OpenSPIM instruments worldwide have been posted on the wiki.5 The number of unpublished systems is unknown, but more systems are certainly under construction. In any case, global interest in OpenSPIM is remarkable. Curious students and researchers use every possible opportunity to get familiar with the system. Experts say that OpenSPIM does not reach the standards of commercially available or sophisticated home-built systems, but due to its significantly lower costs it is widely accessible to a much broader user community. This makes applications possible, for example, for universities in countries that have very limited research budgets and that could not afford other SPIM systems. Because OpenSPIM is open to all and not proprietary, its usefulness in educational contexts is unparalleled. Anyone who buys a light-sheet microscope can use it, but anyone who assembles an OpenSPIM will also understand it! Beyond that, OpenSPIM also makes research more transparent. Experimental results can be understood and reproduced more easily by peers and thus be verified, which contributes to the integrity and authentication of research results.
Whether OpenSPIM will continue to expand or simply remain an exciting niche project remains to be seen. This will substantially depend on whether or not the SPIM community – including the DIY builders and commercial providers – recognize the value of their ongoing commoning and perceive themselves as active commoners. Will they let their project modifications and improvements continue to flow back to the community and contribute to its flourishing?
If the OpenSPIM platform is seen simply as a launch pad for the proprietary “secret projects” of either businesses or solitary nerds, it is quite possible the project will collapse. The community of OpenSPIM enthusiasts might at some point become exhausted by their voluntary efforts, and find it easier to retreat to their own private, proprietary interests. But there are reasons for optimism: In January 2015, the highly respected journal Nature Methods, which is affiliated with the prominent interdisciplinary scientific journal Nature, selected light-sheet microscopy as “Method of the Year 2014.”6 This will provide strong tailwinds for the OpenSPIM endeavor!
Jacques Paysan (Germany) holds a PhD in Neurobiology, and is a commons fan and SPIM Expert.He lives in the Jagst-Valley in Baden-Württemberg.
- The optical axis represents a straight line from object to image through the centers of symmetry of all lenses of a microscope.
- https://www.nobelprize.org/nobel_prizes/chemistry/laureates/1925/press.html
- www.openspim.org
- Editors’ note: See the essay on alternative licensing.
- OpenSPIM should not be confused with customized SPIM systems. Some of the latter are specialized and highly sophisticated systems constructed for specific applications. The costs of such systems can reach hundreds of thousands of euros. In contrast, the costs of an OpenSPIM, depending on its configuration, are typically in the range of tens of thousand euros.
- https://www.nature.com/nmeth/journal/v12/n1/full/nmeth.3251.htm